Development of landslide triggering model and model application
The landslide triggering model I have developed is an extended version of the models introduced in Lehmann and Or [2012] and von Ruette et al. [2013] that were motivated by the concepts of self-organized criticality. A key feature of our modeling approach is the implementation of threshold mechanics where mechanical interactions are represented by conceptual mechanical bonds (interconnecting neighboring soil columns and soil-bedrock interface) with defined strength thresholds. The key difference between the model I have developed and implemented and the models of Lehmann and Or [2012] and von Ruette et al. [2013] lies in the FBM representation of the virtual mechanical bonds. Lehmann and Or [2012] considered bundles consisting of about 10’000 fibers defined by uniform strength distributions and applied analytical solutions for the number of breaking fibers. von Ruette et al. [2013] used a simplified model where the mechanical bonds were represented by a single element and not by an FBM. This version of landslide triggering model opts for small fiber bundles with Weibull distribution for fiber strength to represent the mechanical bonds and compute the number of broken fibers at each computational time step. This modification allows better representation for local failures (e.g., the buckling of load bearing elements in granular materials (force chains), frictional sliding between grains, formation of microcracks in the soil mass, rupture of capillary bridges and breakage of plant roots), and also introduces a certain degree of natural randomness to the system without dramatically increasing the computational burden.
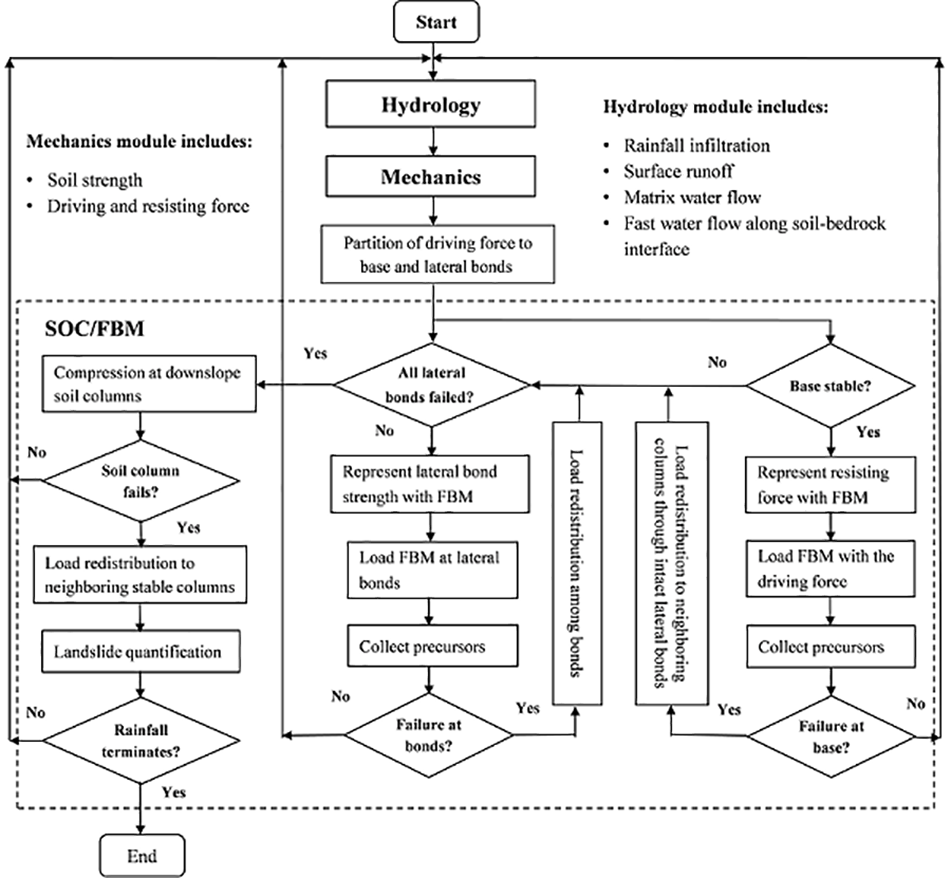
Figure 1: The flow chart of the landslide triggering model for each soil column after adding rainfall volume and computing new water content. The driving and resisting forces at the soil-bedrock interface of each soil column are calculated based on the water content dependent soil strength. The failure of the soil-bedrock interface is followed by load redistribution to its neighboring soil columns via intact mechanical bonds which, in turn, may also fail and transmit the load downslope as compression. When the compressive stress exerting on a soil column exceeds soil compressive strength, the soil column loses its structural integrity and fails and may initiate a chain reaction of failure events. The simulation is terminated when rainfall stops.
Related publication(s):
- Fan, et al, (2015a), Effects of Hydromechanical Loading History and Antecedent Soil Mechanical Damage on Shallow Landslide Triggering, Journal of Geophysical Research: Earth Surface, in press.
- Lehmann, P., and D. Or (2012), Hydromechanical triggering of landslides: From progressive local failures to mass release, Water Resources Research, 48(3).
- Cohen, D., P. Lehmann, and D. Or (2009), Fiber bundle model for multiscale modeling of hydromechanical triggering of shallow landslides, Water resources research, 45(10).
- von Ruette, J., P. Lehmann, and D. Or (2013), Rainfall‐triggered shallow landslides at catchment scale: Threshold mechanics‐based modeling for abruptness and localization, Water Resources Research, 49(10), 6266-6285.
With this landslide triggering model, we systematically quantified effects of spatial variations in soil texture and initial moisture on characteristics of rainfall-induced shallow landslides at the hillslope- and catchment-scales. At the hillslope scale, we model soil spatial variability as univariate distributions of random variables with prescribed probabilit{Western, 2001 #134}y density functions and statistical characteristics such as mean, standard deviation and spatial correlation length. At the catchment scale, soil spatial variability modeled as spatial structure plus stochastic component. Specifically, spatial variable initial water content at the catchment scale was linked to landscape topographic wetness index, whereas the soil texture parameter varied deterministically with soil depth with a spatially correlated stochastic residual. This study has revealed different effects of structured and stochastic soil variability on landslide metrics on elapsed time to landslide triggering and landslide characteristics for similar mean soil property values and identical external boundary conditions (e.g., rainfall).
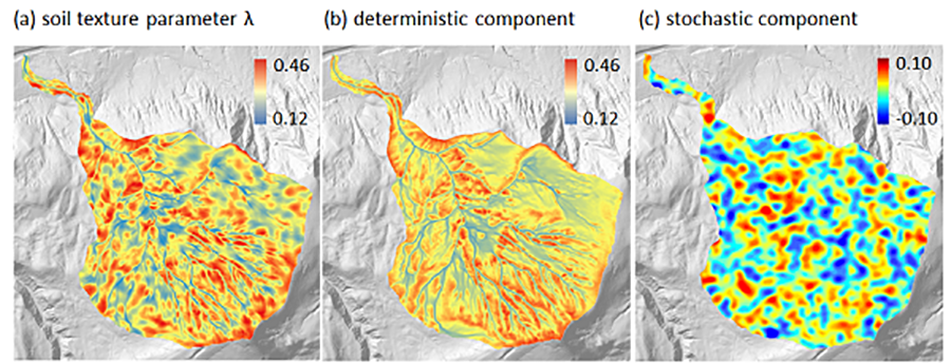
Figure 2: Generation of the spatial distribution of soil texture parameter λ (a) as a composite of the soil depth dependent deterministic component (b) and the stochastic component (c) for Eriz catchment (Switzerland). For the deterministic component, soil texture parameter is negatively correlated to soil depth. The standard deviation and spatial correlation length of the shown stochastic component is 0.030 and 10 m respectively.
Related publication(s):
- Fan, et al, (2015), Effects of Soil Spatial Variability at the Hillslope and Catchment Scales on Characteristics of Rainfall-Induced Landslides, Water Resources Research, under review.
Team: Dani Or, Peter Lehmann, Linfeng Fan
Funding: Competence Center Environment and Sustainability (CCES)
Students: Linfeng Fan
Contact: